top of page
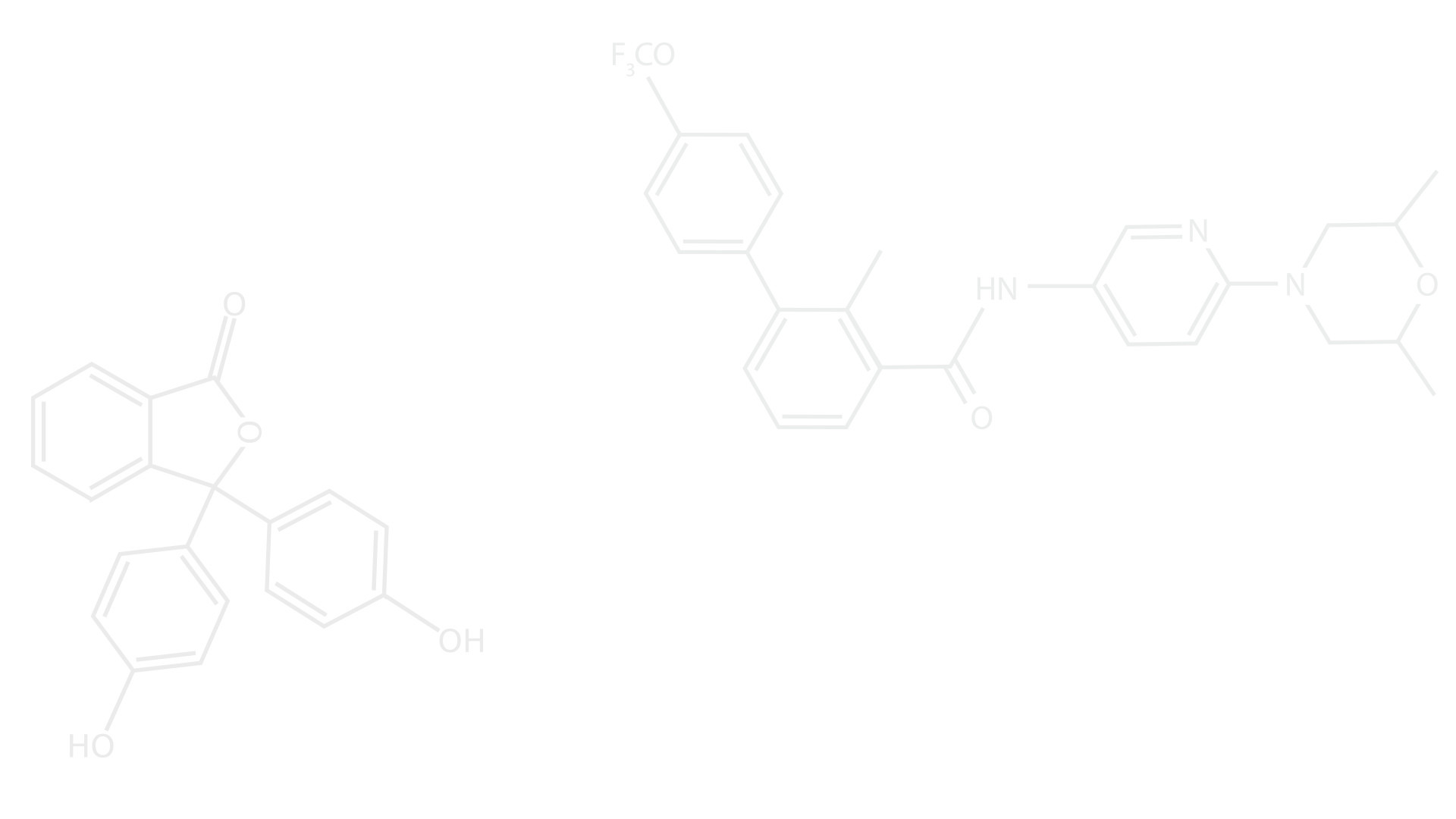
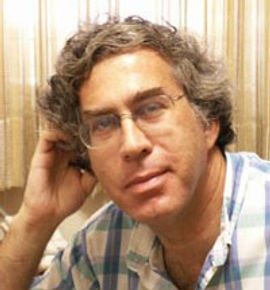
Our group specializes in theoretical and experimental studies of exciting and innovative research fields such as machine learning, extracellular and intracellular stimulations and recordings of neurons in-vitro, synchronization of neural networks and chaotic lasers, physical random number generators and advanced protocols for secure communication.
Recent Publications
Latest videos
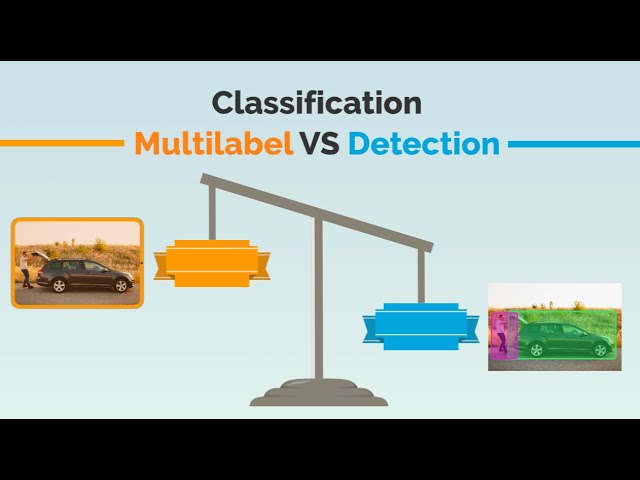
Search video...

03:09
Play Video
Multilabel classification outperforms detection-based technique

03:30
Play Video
The role of delays in brain dynamics

07:46
Play Video
Physics has mislead neuroscience for over two decades

04:29
Play Video
Classification with changing number of objects using deep learning

03:53
Play Video
Advanced confidence AI applications in autonomous vehicles

04:56
Play Video
How does deep learning work?

04:20
Play Video
Can the shallow brain compete with deep learning

02:22
Play Video
Better paths yield better AI
bottom of page